What Is The Success Rate Of Cloning Animals Through Somatic Cell Nuclear Transfer?
- Review
- Open up Access
- Published:
Cloning animals by somatic cell nuclear transfer – biological factors
Reproductive Biological science and Endocrinology volume 1, Article number:98 (2003) Cite this article
Abstract
Cloning by nuclear transfer using mammalian somatic cells has enormous potential application. However, somatic cloning has been inefficient in all species in which live clones have been produced. High abortion and fetal bloodshed rates are commonly observed. These developmental defects have been attributed to incomplete reprogramming of the somatic nuclei by the cloning process. Diverse strategies have been used to amend the efficiency of nuclear transfer, however, significant breakthroughs are yet to happen. In this review we will talk over studies conducted, in our laboratories and those of others, to gain a better agreement of nuclear reprogramming. Considering cattle are a species widely used for nuclear transfer studies, and more laboratories take succeeded in cloning cattle than whatsoever other specie, this review will be focused on somatic prison cell cloning of cattle.
Introduction
Somatic cell cloning (cloning or nuclear transfer) is a technique in which the nucleus (DNA) of a somatic cell is transferred into an enucleated metaphase-2 oocyte for the generation of a new individual, genetically identical to the somatic jail cell donor (Figure ane). The success of cloning an unabridged animal, Dolly, from a differentiated adult mammary epithelial jail cell [1] has created a revolution in science. It demonstrated that genes inactivated during tissue differentiation can be completely re-activated past a process chosen nuclear reprogramming: the reversion of a differentiated nucleus back to a totipotent status. Somatic cloning may exist used to generate multiple copies of genetically elite farm animals, to produce transgenic animals for pharmaceutical protein product or xeno-transplantation [2–v], or to preserve endangered species. With optimization, it as well promises enormous biomedical potential for therapeutic cloning and allo-transplantation [half-dozen]. In add-on to its practical applications, cloning has become an essential tool for studying gene office [7], genomic imprinting [8], genomic re-programming [nine–12], regulation of development, genetic diseases, and gene therapy, also equally many other topics.
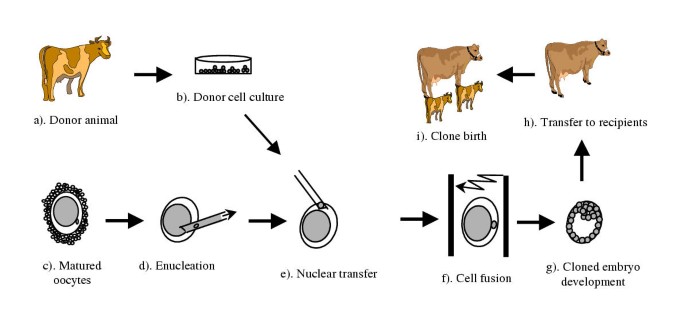
Schematic diagram of the somatic cloning process. Cells are nerveless from donor (a) and cultured in vitro (b). A matured oocyte (c) is and so enucleated (d) and a donor cell is transferred into the enucleated oocyte (e). The somatic cell and the oocyte is then fused (f) and the embryos is allowed to develop to a blastocyst in vitro (g). The blastocyst can then be transferred to a recipient (h) and cloned animals are born after completion of gestation (i).
I of the most difficult challenges faced, however, is cloning'south depression efficiency and high incidence of developmental abnormalities [thirteen–19]. Currently, the efficiency for nuclear transfer is betwixt 0–10%, i.e., 0–10 live births later on transfer of 100 cloned embryos. Developmental defects, including abnormalities in cloned fetuses and placentas, in addition to high rates of pregnancy loss and neonatal death take been encountered by every research team studying somatic cloning. It has been proposed that low cloning efficiency may be largely attributed to the incomplete reprogramming of epigenetic signals [twenty–23].
Factors affecting nuclear reprogramming
Various strategies accept been employed to modify donor cells and the nuclear transfer procedure in attempts to improve the efficiency of nuclear transfer. Most of these efforts are focused on donor cells. These include: a) synchrony of the cell bicycle stage of donor cells [24–26], too as synchrony between donor cells and recipient oocytes [27, 28]; b) using somatic cells from donors of various ages [29–33], tissue origins [26, 34–39], passages [16, 40, 41] and civilisation conditions [42]; c) transfer of stem cells with low levels of epigenetic marks [43–48]; and d) modifying epigenetic marks of donor cells with drugs [49–51]. Although the efficiency of nuclear transfer has been dramatically improved from the initial success charge per unit of one live clone born from 277 embryo transfers [1], none of the aforementioned efforts abolished the common problems associated with nuclear transfer. These observations suggest that further studies on nuclear reprogramming are needed in order to empathise the underlying mechanisms of reprogramming and significantly ameliorate the ability of the differentiated somatic nuclei to be reprogrammed. In the post-obit department, nosotros will discuss several strategies used to improve nuclear transfer efficiencies.
Serum starvation of donor cells
Serum starvation was used in the creation of Dolly and was believed essential to the success of nuclear transfer [1]. Serum starvation induces quiescence of cultured cells, and arrests them at the cell cycle stage of G0. Nigh laboratories that have succeeded with nuclear transfer have utilized a serum starvation treatment. However, there is a argue as to whether inducing quiescence is required for successful nuclear transfer. Cibelli et al. [52] proposed that G0 was unnecessary and that calves could be produced from cycling cells. In his study, actively dividing bovine fibroblasts were used for nuclear transfer and iv calves were born from 28 embryos transferred to eleven recipients. Because 56% of cycling cells in that study were in G1 stage, it is likely that all cloned animals produced in this study were from donor cells at G1 stage. Cells at G2, S or M would not be expected to generate cloned animals in this study considering they are incompatible with the recipient oocytes used. This study demonstrated that cells at G1 stage tin produce alive cloned animals and G0 induction is not essential.
Since the report of Cibelli and colleagues, many laboratories have compared nuclear transfer using donor cells with and without serum starvation. In our study, we used cells from a 17-yr old male Japanese Blackness beef balderdash and found that serum starvation was non required for successful cloning because cloned embryos and animals were produced from cells not subjected to serum starvation (Tabular array i) [16]. Furthermore, serum starvation did non have a beneficial result on the blastocyst evolution of cloned embryos.
In other studies in which serum starvation vs. no starvation were directly compared, evidence was found that both quiescent and proliferating somatic donor cells can be fully reprogrammed afterward nuclear transfer and upshot in viable offspring [25, 26, 29, 53, 54]. Still, it is still debatable which jail cell cycle stage, G0 or G1, result in the best cloning efficiency. Interestingly, Zechkerchenko et al. [53] observed a positive consequence of serum starvation on the efficiency of nuclear transfer using bovine fetal fibroblasts. Although Cho et al. [55] did non detect an improvement in blastocyst rate from any of four different cell types (cumulus, fibroblast, uterine and oviduct epithelial cells). Similar observations were noted by Hills et al. [29] who reported that serum starvation of adult donor cells did non improve development rates of cloned embryos to blastocyst, but when fetal cells were serum-starved, there was a pregnant increase in their blastocyst development. Conversely, Rho et al. [54] found that fetal transgenic lines were non unlike in blastocyst development with or without serum starvation or confluency.
Recently, Kasinathan et al. [25] evaluated methods for generating G0 and G1 prison cell populations and compared their development following cloning. They found that a loftier degree of confluence was more effective than serum starvation for arresting cells in G0, and G1 cells could be obtained using a "milkshake-off" procedure. In this study, no differences in in vitro development were observed between embryos derived from the high-confluence cells (G0) or from the "shaken-off" cells (G1). Yet, when embryos from each handling were transferred into 50 recipients, five calves (10% of embryos transferred) were obtained from embryos derived from the "milk shake-off" cells, whereas no embryos from the confluent cells survived beyond 180 days of gestation. Kasinathan et al. [25] concluded that nuclear transfer donor cell cycle stage is important, particularly effecting belatedly fetal development, and that actively dividing G1 cells support higher development rates than cells in G0. Despite the fact that Kasinathan's study did not produce live clones from G0 cells, a loftier nuclear transfer success charge per unit was obtained by Cho et al. [55] who subjected donor cells to serum starvation and found no improvement in blastocyst development from adult donor cells, but resulted in a 27.3% calving rate.
To further complicate the matter, Wells et al. [26] compared 2 dissimilar types of non-transfected bovine fetal fibroblasts (BFFs) that were synchronized in G0, G1 or different phases within G1. They showed that serum starvation into G0 resulted in a significantly higher pct of viable calves at term than did synchronization in early on G1 or late G1. For transgenic fibroblasts, however, cells selected in G1 showed significantly higher evolution to term of calves and college post-natal survival to weaning, than cells in G0. They suggest that information technology may exist necessary to coordinate donor cell type and prison cell bike phase to maximize overall cloning efficiency.
In summary, it is clear that quiescence is non necessary for the success of nuclear transfer because cells not subjected to serum starvation can too produce alive clones. Fifty-fifty so, it remains unclear which prison cell cycle stage, G0 or G1, imparts a higher nuclear transfer efficiency. This question will go along to be debated until large-scale nuclear transfer studies can exist conducted.
Cloning competence of various somatic cell types
Many somatic prison cell types, including mammary epithelial cells, ovarian cumulus cells, fibroblast cells from skin and internal organs, diverse internal organ cells, Sertoli cells [38, 56], macrophage [56] and blood leukocytes [34, 35] have been successfully utilized for nuclear transfer. A clear consensus, however, has non however been reached as to the superior somatic cell type for nuclear transfer. This is due in part to the fact that dissimilar laboratories employ various procedures; and cell culture, nuclear transfer, and micromanipulation all require critical technical skills. In lodge to brand these comparisons valid, the procedures and techniques used, too equally the skill of lab personnel, must exist identical for each donor animate being and cell type. To compare the competence of unlike jail cell types for reprogramming past cloning, we avoided animate being variation by looking at the cloning competence of three cell types: ovarian cumulus, mammary epithelial and skin fibroblast cells, all from the aforementioned donor animal, a 13-yr-old aristocracy diary cow.
The power of donor cells to be reprogrammed was assessed by the development of cloned embryos in vitro and by the nascency of cloned calves following embryo transfer. As shown in Tables 2 and three, although no differences were detected in the cleavage rates of embryos from three different cell types, cumulus cells produced the highest rate of blastocyst development in this study and resulted in 6 full-term cloned calves. Furthermore, iv out of the 6 calves derived from cumulus cells survived and were however healthy at nearly 4 years of age (Table three). In dissimilarity, the poorest in vitro development, and no total-term survival, was obtained with mammary epithelial cells. Skin fibroblast cells resulted in an intermediate rate of in vitro development and gave rise to 4 full-term cloned calves.
Our results showed that the donor cell type can significantly affect embryo development in vitro besides every bit in vivo. Cumulus cells proved to be the well-nigh constructive jail cell type for somatic cloning co-ordinate to both the in vitro development test as well as total-term survival. These results advise that Deoxyribonucleic acid from cumulus cells is more effectively reprogrammed following nuclear transfer. Our results agreed with those obtained in mice [57] where they compared the nuclear transfer efficiency of neuronal, Sertoli and cumulus cells, and obtained the best alive nascency rate from cumulus cell-derived cloned embryos. Furthermore, it was reported that cumulus prison cell-derived cloned mice do not accept widespread dysregulation of imprinting [23]. Kato et al. [xv, 36] compared cells from the liver, testis, skin, ear, along with cumulus and oviductal cells and concluded that cumulus and oviduct epithelial cells are the most suitable for nuclear donors. Evidence supporting the superiority of cumulus cells for nuclear transfer too comes from the study of Forsberg et al. [58] who conducted big numbers of embryo transfer in cattle. It was shown that cumulus cells gave an overall xv.ii% calving rate, while fetal genital ridge cells, and fibroblast cells produced a 9% calving rate. Adult fibroblast cells, in this study, gave the lowest calving rate of only 5%.
In summary, among the somatic cell types tested, the consensus from numerous laboratories is that cumulus cells give the highest cloning efficiency and outcome in the least number of abnormalities in cloned animals.
Effect of donor age
By using a design like to the donor cell blazon comparing, we studied the cloning efficiency of fibroblast cells from donors of different ages. We found that cells from fetuses and newborn animals were more efficient in nuclear transfer. Even so, when cells from developed animals were used, little changes were observed in the cloning efficiency of cells from cattle varying in age from 2 to16-years-old (Table iv).
Similarly, Renard et al. [31], Hills et al. [29] and Wakayama and Yanagimachi [56] also reported that development rates of somatic cloned embryo remained similar regardless of donor age. Still, Kato et al. [36] noted that clones derived from adult cells frequently aborted in the later stages of pregnancy, and calves developing to term showed a higher number of abnormalities than did those derived from newborn or fetal cells. Forsberg et al. [58] transferred a big number of cloned embryos in cattle. They also ended that, in general, embryos cloned from fetal cells produced higher pregnancy and calving rates than those from adult cells.
In conclusion, it appears that cells from fetuses, likewise equally aged adults, tin lead to comparable blastocyst development of cloned embryos. Withal, fetal cells may be better than developed cells in producing healthy live births. This might be due to the fact that the somatic cells of adult animals have accumulated more genetic mutations/are more terminally differentiated than fetal cells, and are thus more probable to fail at full term evolution.
Issue of jail cell culture duration (passage numbers)
Our grouping was the start to directly compare passage event of donor cells on the outcome of nuclear transfer [16]. In our study, we found that cells of afterwards passages (up to xv) could too support clone development to full term (Tabular array five).
Comparable to our findings were those of Arat et al. [40] who established a chief cell line from granulosa cells and transfected them with the green fluorescence protein (GFP) gene. Non-transfected cells were used for cloning between passage ten and 15 as either serum-starved or serum-fed donor cells. There were no differences in evolution to the blastocyst stage for nuclear transfer embryos from transfected or non-transfected or from serum-starved or serum-fed cells. Blastocyst evolution rates of embryos produced from donor cells at passage 15, all the same, were significantly college than those produced with cells at passage ten, xi, and thirteen. Developmental competence of after passages, up to 16 [54] and equally loftier every bit 36, from fibroblast from a cloned fetus [41], accept also been reported.
The demonstration that afterward passages can support clone development is essential for utilizing somatic cloning for gene-knockout studies, in which single cells must be clonally expanded to generate sufficient cells for nuclear transfer [7]. These afore-mentioned studies suggest that cells of higher passages were receptive to nuclear reprogramming. Additional support for this hypothesis comes from a recent study past Enright et al. [59] who showed that cells of later on passages incorporate less epigenetic modifications, i.due east., their histones are more acetylated than in earlier passages. This observation agrees with an earlier notion that in vitro culture of cells can induce expression of genes that were not expressed before civilization [60, 61]. Furthermore, Hills et al. [62] reported that a greater proportion of late passage cells (passage eighteen), vs. earlier passage cells (passage 2), were establish to be in G0/G1 whether or non they were in serum-starved culture conditions.
Effect of modification of pre-existing epigenetic marks in donor cells
Histone acetylation and Dna methylation are heritable modifications of the chromatin that do not involve changes in gene sequences (epigenetic signals). These epigenetic modifications are believed responsible for the derivation of diverse cell types with the same genetic makeup. In natural reproduction, relatively depression levels of DNA methylation be in the gametes, which are further de-methylated during early embryo evolution [63, 64]. With nuclear transplantation, the somatic donor nucleus carries the specific epigenetic modifications of its tissue type, which must be erased during nuclear reprogramming. Therefore, the levels of epigenetic modification existing in donor cells may affect their reprogrammability post-obit nuclear transfer. As discussed earlier, a discrepancy in the donor cell's susceptibility to reprogramming has been observed betwixt different cell types, resulting in differences in vitro and in vivo development of cloned embryos. Therefore, treating donor cells with pharmacological agents to remove some epigenetic marks prior to nuclear transfer may improve the ability of the donor cells to be fully reprogrammed by the recipient karyoplast.
Two reagents have been widely used for the alteration of the levels of epigenetic modification of somatic cells. Trichostatin A (TSA) and 5-aza-deoxy-cytadine (five-aza-dC) have been found to increase histone acetylation and decrease Deoxyribonucleic acid methylation, respectively. These changes accept been associated with increases of gene expression. Recently, we conducted studies in which the pre-existing epigenetic marks in donor cells were reduced by these drugs [49]. Nosotros found that global epigenetic marks in donor cells can be modified by handling with TSA or 5-aza-dC. Unfortunately, treating donor cells with 5-aza-dC reduced blastocyst formation of cloned embryos. Previously, Jones et al. [l] and Zhou et al. [51] treated bovine fetal fibroblast cells and mouse stem cells with much college doses of five-aza-C (one or 5 μm) and also plant that blastocyst development of cloned embryos were reduced. The consensus from these studies [49–51] suggests that lowering the levels of DNA methylation in donor cells does not always meliorate development of cloned embryos. At high concentrations, v-aza-dC may have been cytotoxic to the donor cells. Additionally, prolonged handling at a lower concentration, as was the case in our study, may take acquired severe hypo-methylation, and resulted in disrupted expression of essential genes important for embryo development. Therefore, further experiments are required to test the effects of lower concentrations and shorter durations of 5-aza-dC handling on donor cells.
Treating donor cells with TSA, by contrast, significantly improved development of cloned embryos. Previous reports indicated that handling of mouse stem cells with TSA reduced evolution of cloned embryos [51]. The differences between these findings may be due to the variation in the concentrations of TSA used. Prior to nuclear transfer, we treated donor cells with a broad range of TSA concentrations and identified the lowest concentration capable of inducing histone hyperacetylation (ane.25 μM). The everyman concentration tested (0.08 μM), did non cause hyperacetylation, but resulted in observable changes in prison cell morphology, similar to those described previously [65]. It was this lower concentration of TSA (0.08 μM) that improved development of cloned embryos in our study, while the higher concentration (1.25 μM) inhibited embryo development. The detrimental consequence of a higher dose of TSA on embryo development may exist explained past the fact that treatment of cells with high concentrations of TSA causes chromatin breaks and apoptosis [66].
Conclusion
Somatic jail cell cloning by nuclear transfer is a relatively new applied science with many potential applications. However, at the current stage of development, the reprogramming of epigenetic inheritance by nuclear transfer is withal incomplete. Further efforts and new paradigms are needed to perfect this technology and extend it to its fullest potential.
References
-
Wilmut I, Schnieke AE, McWhir J, Kind AJ, Campbell KHS: Viable offspring derived from fetal and adult mammalian cells. Nature. 1997, 385: 810-813. 10.1038/385810a0.
-
Anderson GB, Seidel GE: Cloning for profit. Science. 1998, 280: 1400-1401. 10.1126/science.280.5368.1400.
-
Polejaeva IA, Campbell KHS: New advances in somatic cell nuclear transfer: Application in transgenesis. Theriogenology. 2000, 53: 117-126. 10.1016/S0093-691X(99)00245-9.
-
Robl J: Development and application of applied science for large scale cloning of cattle. Theriogenology. 1999, 51: 499-508. x.1016/S0093-691X(98)00243-Ten.
-
Stice SL, Robl JM, Ponce de Leon FA, Jerry J, Golueke PG, Cibelli JB, Kane JJ: Cloning: new breakthroughs leading to commercial opportunities. Theriogenology. 1998, 49: 129-138. 10.1016/S0093-691X(97)00407-X.
-
Lanza RP, Cibelli JB, West Md: Human therapeutic cloning. Nat Med. 1999, five: 975-977. x.1038/12404.
-
Capecchi MR: How close are we to implementing factor targeting in animals other than the mouse?. Proc Natl Acad Sci USA. 2000, 97: 956-957. 10.1073/pnas.97.3.956.
-
Solter D: Imprinting. Int J Dev Biol. 1998, 42: 951-954.
-
De Sousa PA, Winger Q, Hill JR, Jones Chiliad, Watson AJ, Westhusin ME: Reprogramming of fibroblast nuclei after transfer into bovine oocytes. Cloning. 1999, 1: 63-69. 10.1089/15204559950020102.
-
Munsie MJ, Michalska AE, O'Brien CM, Trounson AO, Pera MF, Mountford PS: Isolation of pluripotent embryonic stalk cells from reprogrammed adult mouse somatic nuclei. Curr Biol. 2000, 10: 989-992. 10.1016/S0960-9822(00)00648-5.
-
Surani MA: Reprogramming of genome office through epigenetic inheritance. Nature. 2001, 414: 122-128. 10.1038/35102186.
-
Winger QA, Hill JR, Shin T, Watson AJ, Kraemer DC, Westhusin ME: Genetic reprogramming of lactate dehydrogenase, citrate synthase, and phosphofructokinase mRNA in bovine nuclear transfer embryos produced using bovine fibroblast prison cell nuclei. Mol Reprod Dev. 2000, 56: 458-464. ten.1002/1098-2795(200008)56:4<458::Aid-MRD3>iii.3.CO;2-C.
-
Garry FB, Adams R, McCann JP, Odde KG: Postnatal characteristics of calves produced by nuclear transfer cloning. Theriogenology. 1996, 45: 141-152. 10.1016/0093-691X(95)00363-D.
-
Colina JR, Roussel AJ, Cibelli JB, Edwards JF, Hooper NL, Miller MW, Thompson JA, Looney CR, Westhusin ME, Robl JM, Stice SL: Clinical and pathologic features of cloned transgenic calves and fetuses (13 instance studies). Theriogenology. 1999, 51: 1451-1465. 10.1016/S0093-691X(99)00089-8.
-
Kato Y, Tani T, Sotomaru Y, Kurokawa One thousand, Kato J, Doguchi H, Yasue H, Tsunoda Y: Eight calves cloned from somatic cells of a single adult. Scientific discipline. 1998, 282: 2095-2098. 10.1126/science.282.5396.2095.
-
Kubota C, Yamakuchi H, Todoroki J, Mizoshita Yard, Tabara Northward, Hairdresser Yard, Yang 10: Six cloned calves produced from developed fibroblast cells afterwards long-term culture. Proc Natl Acad Sci Usa. 2000, 97: 990-995. 10.1073/pnas.97.3.990.
-
Renard J-P, Chastnat Due south, Chesne P, Richard C, Marchal J, Cordonnier N, Chavatte P, Vignon X: Lymphoid hypoplasia and somatic cloning. Lancet. 1999, 353: 1489-1491. x.1016/S0140-6736(98)12173-half-dozen.
-
Walker SK, Hartwich KM, Seamark RF: The product of unusually big offspring post-obit embryo manipulation: concepts and changes. Theriogenology. 1996, 45: 111-120. x.1016/0093-691X(95)00360-K.
-
Young LE, Sinclear KD, Wilmut I: Large offspring syndrome in cattle and sheep. Rev Reprod. 1998, three: 155-163. ten.1530/revreprod/3.3.155.
-
Bourc'his D, Le Bourhis D, Patin D, Niveleau A, Comizzoli P, Renard J-P, Viegas-Pe'quignot Due east: Delayed and incomplete reprogramming of chromosome methylation patterns in bovine cloned embryos. Curr Biol. 2001, eleven: 1542-1546. 10.1016/S0960-9822(01)00480-eight.
-
Dean Westward, Santos F, Stojkovic M, Zakhartchenko 5, Walter J, Wolf East, Reik W: Conservation of methylation reprogramming in mammalian evolution: aberrant reprogramming in cloned embryos. Proc Natl Acad Sci USA. 2001, 98: 13734-13738. 10.1073/pnas.241522698.
-
Kang Y-M, Koo D-B, Park J-S, Choi Y-H, Lee Chiliad-Grand, Han Y-M: Aberrant methylation of donor genome in cloned bovine embryos. Nat Genet. 2001, 28: 173-177. 10.1038/88903.
-
Rideout WM, Eggan K, Jaenisch R: Nuclear cloning and epigenetic reprogramming of the genome. Science. 2001, 293: 1093-1098. 10.1126/science.1063206.
-
Gibbons J, Arat S, Rzucidlo J, Miyoshi K, Waltenburg R, Respess D, Venable A, Stice S: Enhanced survivability of cloned calves derived from roscovitine-treated adult somatic cells. Biol Reprod. 2002, 66: 895-900.
-
Kasinathan P, Knott JG, Wang Z, Jerry DJ, Robl JM: Production of calves from G1 fibroblasts. Nat Biotechnol. 2001, 19: 1176-1178. 10.1038/nbt1201-1176.
-
Wells DN, Laible G, Tucker FC, Miller AL, Oliver JE, Xiang T, Forsyth JT, Berg MC, Cockrem K, 50'Huillier PJ, Tervit Hr, Oback B: Coordination between donor prison cell blazon and prison cell wheel stage improves nuclear cloning efficiency in cattle. Theriogenology. 2003, 59: 45-59. 10.1016/S0093-691X(02)01273-six.
-
Campbell KH, Loi P, Cappai P, Wilmut I: Improved evolution to blastocyst of ovine nuclear transfer embryos reconstructed during the presumptive Due south-stage of enucleated activated oocytes. Biol Reprod. 1994, 50: 1385-1393.
-
Du F, Sung L-Y, Tian XC, Yang Ten: Differential Cytoplast Requirement for Embryonic and Somatic Cell Nuclear Transfer in Cattle. Mol Reprod Dev. 2002, 63: 183-191. 10.1002/mrd.10172.
-
Hill JR, Winger QA, Long CR, Looney CR, Thompson JA, Westhusin ME: Development rates of male bovine nuclear transfer embryos derived from developed and fetal cells. Biol Reprod. 2000, 62: 1135-1140.
-
Kasinathan P, Knott JG, Moreira PN, Burnside Equally, Jerry DJ, Robl JM: Outcome of fibroblast donor prison cell age and prison cell cycle on evolution of bovine nuclear transfer embryos in vitro. Biol Reprod. 2001, 64: 1487-1493.
-
Renard JP: Chromatin remodeling and potential for full term development of cloned embryos. In Proceedings of Transgenic Animals in Research. Briefing Proceedings of Transgenic Animal Research Conference: Aug, 1999. 1999, Tahoe Urban center, CA, xv-
-
Tian XC, Xu J, Yang X: Normal telomere lengths found in cloned cattle. Nat Genet. 2000, 26: 272-273. 10.1038/81559.
-
Xue F, Tian XC, Kubota C, Du F, Taneja 1000, Dinnyes A, Dai Y, Levine H, Pereira LV, Yang X: Aberrant X-Chromosome inactivation in deceased cattle derived from somatic cloning. Nat Genet. 2002, 31: 216-220. x.1038/ng900.
-
Galli C, Duchi R, Moor RM, Lazzari Thousand: Mammalian leukocytes contain all the genetic information necessary for the development of a new private. Cloning. 1999, one: 161-170. 10.1089/15204559950019924.
-
Hochedlinger Thousand, Jaenisch R: Monoclonal mice generated by nuclear transfer from mature B and T donor cells. Nature. 2002, 415: 1035-1038. 10.1038/nature718.
-
Kato Y, Tani T, Tsunoda Y: Cloning of calves from various somatic prison cell types of male person and female adult, newborn and fetal cows. J Reprod Fertil. 2000, 120: 231-237. x.1530/reprod/120.ii.231.
-
Miyashita N, Shiga Yard, Yonai M, Kaneyama G, Kobayashi S, Kojima T, Goto Y, Kishi Yard, Aso H, Suzuki T, Sakaguchi One thousand, Nagai T: Remarkable differences in telomere lengths among cloned cattle derived from different cell types. Biol Reprod. 2002, 66: 1649-1655.
-
Ogura A, Inoue K, Ogonuki N, Noguchi A, Takano K, Nagano R, Suzuki O, Lee J, Ishino F, Matsuda J: Production of male cloned mice from fresh, cultured, and cryopreserved immature Sertoli cells. Biol Reprod. 2000, 62: 1579-1584.
-
Shiga K, Fujita T, Hirose M, Sasae Y, Nagai T: Production of calves by transfer of nuclei from cultured somatic cells obtained from Japanese blackness bulls. Theriogenology. 1999, 52: 527-535. x.1016/S0093-691X(99)00149-ane.
-
Arat South, Rzucidlo SJ, Gibbons J, Miyoshi M, Stice SL: Product of transgenic bovine embryos by transfer of transfected granulosa cells into enucleated oocytes. Mol Reprod Dev. 2001, 60: 20-26. 10.1002/mrd.1057.
-
Liu L, Shin T, Pryor JH, Kraemer D, Westhusin M: Regenerated bovine fetal fibroblasts support high blastocyst development following nuclear transfer. Cloning. 2001, 3: 51-58. x.1089/15204550152475554.
-
Zakhartchenko Five, Alberio R, Stojkovic One thousand, Prelle K, Schernthaner W, Stojkovic P, Wenigerkind H, Wanke R, Duchler One thousand, Steinborn R, Mueller M, Brem GE: Developed cloning in cattle: potential of nuclei from a permanent cell line and from principal cultures. Mol Reprod Dev. 1999, 54: 264-272. 10.1002/(SICI)1098-2795(199911)54:three<264::AID-MRD7>3.0.CO;2-Y.
-
Amano T, Kato Y, Tsunoda Y: Full-term development of enucleated mouse oocytes fused with embryonic stalk cells from unlike cell lines. Reproduction. 2001, 121: 729-733. ten.1530/reprod/121.5.729.
-
Eggan K, Akutsu H, Loring J, Jackson-Grusby L, Klemm Thousand, Rideout WM, Yanagimachi R, Jaenisch R: Hybrid vigor, fetal overgrowth, and viability of mice derived by nuclear cloning and tetraploid embryo complementation. Proc Natl Acad Sci USA. 2001, 98: 6209-6214. 10.1073/pnas.101118898.
-
Humpherys D, Eggan Chiliad, Akutsu H, Hochedlinger G, Rideout WM, Biniszkiewicz D, Yanagimachi R, Jaenisch R: Epigenetic instability in ES cells and cloned mice. Science. 2001, 293: 95-97. 10.1126/science.1061402.
-
Kato Y, Rideout III W, Hilton K, Barton SC, Tsunoda Y, Surani MA: Developmental potential of mouse primordial germ cells. Development. 1999, 126: 1823-1832.
-
Wakayama T, Rodriguez I, Perry ACF, Yanagimachi R, Mombaerts P: Mice cloned from embryonic stem cells. Proc Natl Acad Sci U.s.a.. 1999, 96: 14984-14989. x.1073/pnas.96.26.14984.
-
Zhou Q, Jouneau A, Brochard V, Adenot P, Renard JP: Developmental potential of mouse embryos reconstructed from metaphase embryonic stem prison cell nuclei. Biol Reprod. 2001, 65: 412-419.
-
Enright BP, Kubota C, Yang X, Tian XC: Epigenetic characteristics and development of embryos cloned from donor cells treated by Trichostatin A or 5-aza-two'-deoxycytidine. Biol Reprod. 2003, 69: 896-903.
-
Jones KL, Loma J, Shin TY, Lui L, Westhusin M: Deoxyribonucleic acid hypomethylation of karyoplasts for bovine nuclear transplantation. Mol Reprod Dev. 2001, sixty: 208-213. ten.1002/mrd.1079.
-
Zhou Q, Baquir South, Brochard V, Smith LC, Renard JP: Donor nuclei are non well reprogrammed by nuclear transfer procedure. Biol Reprod. 2002, 66 (suppl i): 237-238. (s345)
-
Cibelli P, Stice SL, Golueke PJ, Kane JJ, Jerry J, Blackwell C, deLeon FAP, Robl JM: Cloned transgenic calves produced from non-quiescent fetal fibroblasts. Science. 1998, 280: 1256-1258. 10.1126/scientific discipline.280.5367.1256.
-
Zakhartchenko V, Durcova-Hills One thousand, Stojkovic M, Schernthaner Due west, Prelle Yard, Steinborn R, Muller M, Brem Chiliad, Wolf E: Effects of serum starvation and re-cloning on the efficiency of nuclear transfer using bovine fetal fibroblasts. J Reprod Fertil. 1999, 115: 325-331.
-
Roh S, Shim H, Hwang WS, Yoon JT: In vitro evolution of green fluorescent protein (GFP) transgenic bovine embryos later nuclear transfer using different prison cell cycles and passages of fetal fibroblasts. Reprod Fertil Dev. 2000, 12: i-6. ten.1071/RD00021.
-
Cho JK, Lee BC, Park JI, Lim JM, Shin SJ, Kim KY, Lee BD, Hwang WS: Development of bovine oocytes reconstructed with different donor somatic cells with or without serum starvation. Theriogenology. 2002, 57: 1819-1828. 10.1016/S0093-691X(01)00699-ix.
-
Wakayama T, Yanagimachi R: Mouse cloning with nucleus donor cells of unlike historic period and type. Mol Reprod Dev. 2001, 58: 376-383. x.1002/1098-2795(20010401)58:4<376::Assist-MRD4>3.0.CO;2-L.
-
Wakayama T, Perry Air-conditioning, Zuccotti Yard, Johnson KR, Yanagimachi R: Total-term development of mice from enucleated oocytes injected with cumulus cell nuclei. Nature. 1998, 394: 369-374. 10.1038/28615.
-
Forsberg EJ, Strelchenko NS, Augenstein ML, Betthauser JM, Childs LA, Eilertsen KJ, Enos JM, Forsythe TM, Golueke PJ, Koppang RW, Lange G, Lesmeister TL, Mallon KS, Mell GD, Misica PM, Pace MM, Pfister-Genskow G, Voelker GR, Watt SR, Bishop MD: Production of cloned cattle from in vitro systems. Biol Reprod. 2002, 67: 327-333.
-
Enright BP, Jeong BS, Yang X, Tian XC: Epigenetic Characteristics of Bovine Donor Cells for Nuclear Transfer: Levels of Histone Acetylation. Biol Reprod. 2003
-
Hirayu H, Dere WH: Rapoport B. Initiation of normal thyroid cells in primary civilisation associated with enhanced c-myc messenger ribonucleic acid levels. Endocrinology. 1987, 120: 924-928.
-
Baker TK, Carfagna MA, Gao H, Dow ER, Li Q, Searfoss GH, Ryan TP: Temporal gene expression analysis of monolayer cultured rat hepatocytes. Chem Res Toxicol. 2001, 14: 1218-1231. ten.1021/tx015518a.
-
Hill JR, Winger QA, Burghardt RC, Westhusin ME: Bovine nuclear transfer embryo development using cells derived from a cloned fetus. Anim Reprod Sci. 2001, 67: 17-26. 10.1016/S0378-4320(01)00106-3.
-
Mayer W, Niveleau A, Walter J, Fundele R, Haaf T: Demethylation of the zygotic paternal genome. Nature. 2000, 403: 501-502.
-
Oswald J, Engemann South, Lane North, Mayer W, Olek A, Fundele R, Dean Due west, Reik Westward, Walter J: Active demethylation of the paternal genome in the mouse zygote. Curr Biol. 2000, 10: 475-478. 10.1016/S0960-9822(00)00448-6.
-
Hoshikawa Y, Kwon HJ, Yoshida M, Horinouchi S, Beppu T: Trichostatin A induces morphological changes and gelsolin expression by inhibiting histone deacetylase in homo carcinoma jail cell lines. Exp Prison cell Res. 1994, 214: 189-197. 10.1006/excr.1994.1248.
-
Nakajima H, Kim YB, Terano H, Yoshida Chiliad, Horinouchi S: FR90 a Strong Antitumor Antibiotic, Is a Novel Histone deacetylase inhibitor. Expt Cell Res. 1228, 241: 126-133. 10.1006/excr.1998.4027.
Acknowledgement
The authors would like to give thanks Marina Julian for careful reading and editing this manuscript.
Writer information
Authors and Affiliations
Corresponding author
Authors' original submitted files for images
Rights and permissions
About this commodity
Cite this article
Tian, X.C., Kubota, C., Enright, B. et al. Cloning animals by somatic cell nuclear transfer – biological factors. Reprod Biol Endocrinol ane, 98 (2003). https://doi.org/10.1186/1477-7827-ane-98
-
Received:
-
Accepted:
-
Published:
-
DOI : https://doi.org/10.1186/1477-7827-one-98
Keywords
- nuclear transfer
- donor jail cell types
- donor age
- serum starvation
- jail cell passage
Source: https://rbej.biomedcentral.com/articles/10.1186/1477-7827-1-98#:~:text=One%20of%20the%20most%20difficult,transfer%20of%20100%20cloned%20embryos.
Posted by: winklerwhadminvabot.blogspot.com
0 Response to "What Is The Success Rate Of Cloning Animals Through Somatic Cell Nuclear Transfer?"
Post a Comment